Modeling of Turbulence and Turbulent Reactive Flows
Most flows involving human made devices or flows in the environment are turbulent and involve a large range of length and time scales. Consequently such flows are expensive to solve numerically. To reduce the computational burden, methods are applied that solve only for a fraction of these scales but require turbulence models to incorporate effects that result from neglected scales. Most important are Reynolds-averaged Navier-Stokes (RANS) models, large eddy simulation (LES) and probability density function (PDF) methods.
Worldwide, more than 80% of the consumed energy is converted by burning fossil fuels. Therefore, improving emission rates and efficiency of combustion devices automatically has a significant impact on our environment and is of crucial importance. To achieve such improvements, however, the capability of accurately predicting the governing physical processes (which involve turbulence-reaction interaction) is essential. A modeling approach, which proved to be very general and powerful, is based on solving a joint PDF transport equation. Opposed to other approaches, such PDF methods require no model for turbulent convection and there exist no closure issues with averaging the reaction source terms.
Turbulent Combustion Modeling
To combat climate change by reducing greenhouse gases, combustion technologies relying on bio-fuels or oxy-coal combustion need to be implemented. Particles in pulverized coal burners or droplets in combustion chambers of Diesel engines resp. gas turbines self-organize into clusters in turbulent flows. Turbulence in these devices enhances mixing of fuel and oxidizer to maximize the rate of chemical/thermal energy conversion through combustion. While at the level of single droplets or particles, this energy conversion process has been investigated in great detail, studies into turbulence-induced particle or droplet clustering under controlled conditions have been scarce. Moreover, modeling efforts that reproduce clustering effects in computationally low-cost simulations, that do not resolve small-scale turbulence, are limited.
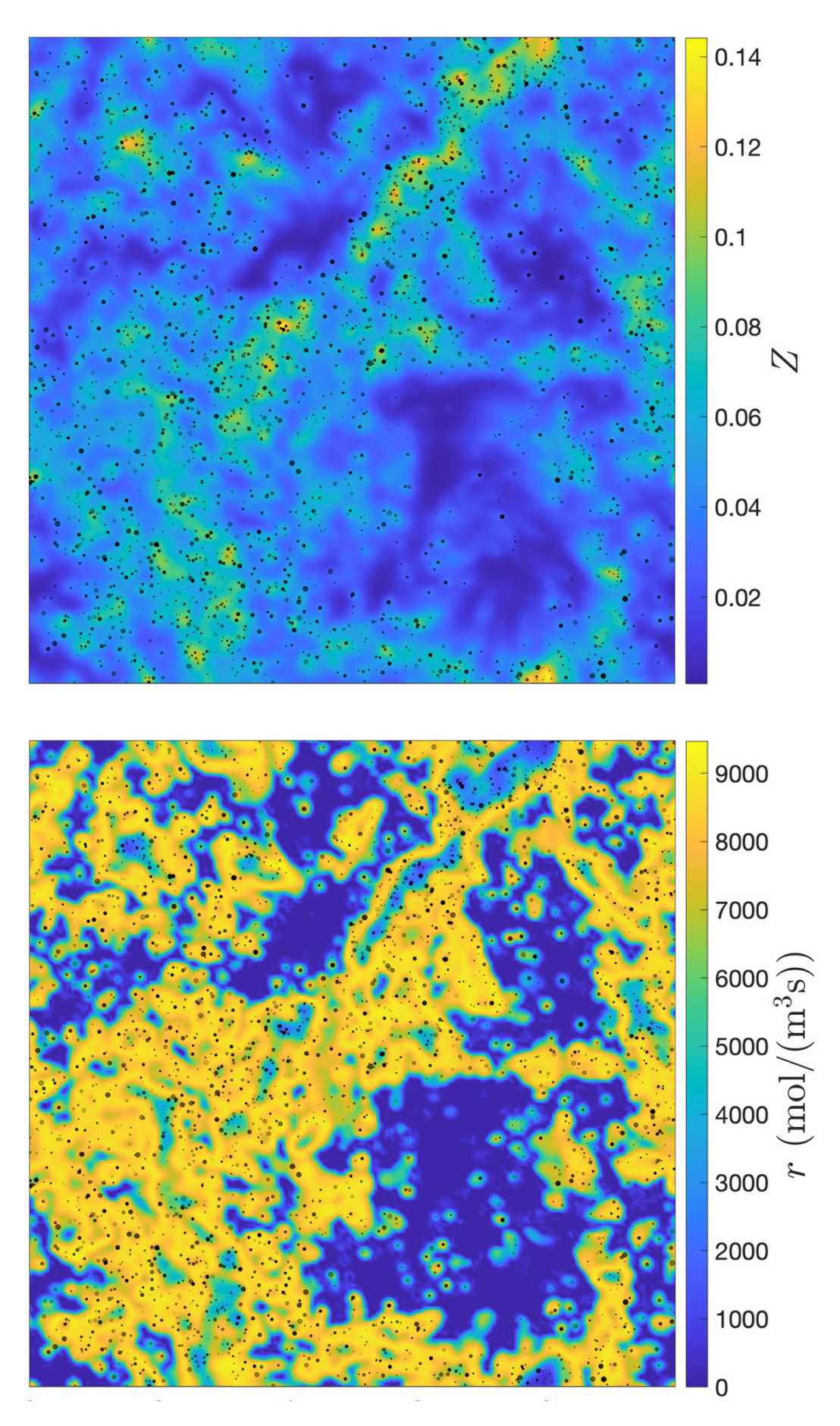
To address these gaps, we have been conducting direct numerical simulations that resolve all turbulence scales of droplet-or particle-laden turbulence for a range of governing parameters [external page Weiss et al. 2018, external page 2019, external page 2020, and external page 2021]. As depicted in the figure resulting from one of our simulations, droplets evaporate and burn (reaction source r) under different conditions depending on whether they reside in clusters or not (fuel mixture fraction Z). In addition to investigating particle/droplet-group effects in turbulent combustion, we have been devising models that allow the reproduction of droplet evaporation resp. particle volatilization dynamics in simulations missing information about the structure of small-scale turbulence [external page Jenny et al. 2012] and [external page Giddey et al. 2018]. An earlier contribution from our group is dealing with the modeling of turbulence modulation due to droplet or particles [external page Meyer 2012].
In connection with PDF methods for gas-phase turbulent reactive flows, we have devised a new model, where reaction and mixing are treated simultaneously [external page Hegetschweiler et al. 2012], a mixing model conditional on velocities [external page Meyer and Jenny 2013], and a PDF model for premixed combustion based on flame surface density propagation [external page Zoller et al. 2013].
Temporal Large Eddy Simulation
A novel temporal large-eddy simulation (TLES) model with direct deconvolution was devised. In contrast to previous approaches such as the temporal approximate deconvolution model (TADM), the non-filtered velocity field is recovered using the differential form of the filter operation rather than from a truncated series expansion of the inverse filter operator. This direct deconvolution is used to obtain formal closure of an analytic evolution equation of the temporal residual-stress tensor. Thus, the temporal direct deconvolution model (TDDM) has advantages relative to the TADM in being both more accurate and requiring less computational effort [external page Oberle et al. 2020]. Results demonstrate a significant improvement. It has been found that TDDM with secondary regularization allows to reconstruct more of the energy containing dynamics of turbulent flows than previous LES or TLES models.
Hybrid LES/RANS Modeling Framework
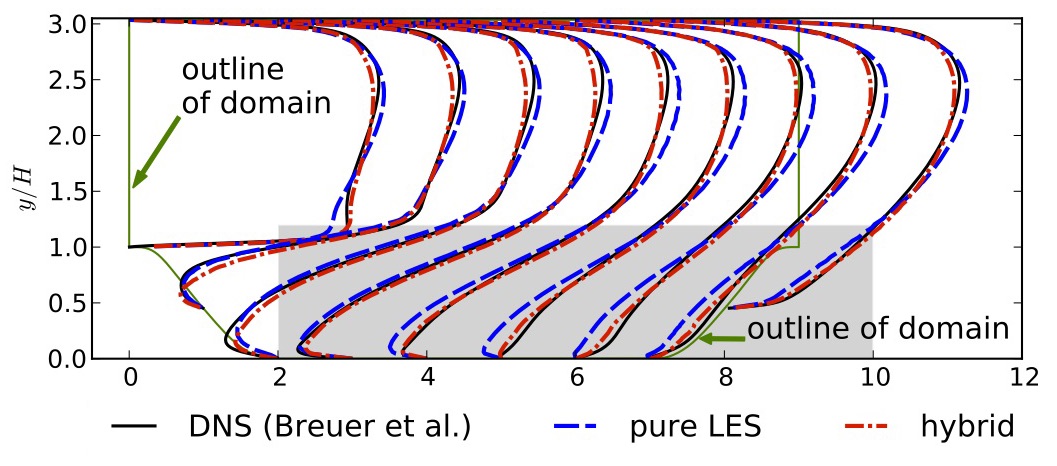
While LES is a very powerful approach to model turbulent flows, it is not yet widely used in industrial workflows. This is mainly due to the high computational cost, which is Reynolds number dependent, if wall turbulence is involved. Another difficulty is the choice of an appropriate computational grid. Motivated by these shortcomings, various hybrid LES/RANS methods have been proposed. A major challenge thereby is to determine the RANS and LES regions and to provide valid boundary conditions between them. We follow a new approach which is based on simultaneous LES and RANS simulations, which are coupled via forcing terms to ensure internal consistency. This allows to overcome most of the problems at RANS/LES interfaces, which are intrinsic in other hybrid methods.
Xiao, Jenny, and coworkers [external page 2012, external page 2013, external page 2014, and external page 2017] have devised a consistent dual-mesh hybrid LES/RANS framework for turbulence modeling. The filtered and Reynolds averaged Navier–Stokes (RANS) equations are solved simultaneously in the whole domain on their respective meshes. Consistency between the two solutions is achieved in terms of mean velocity, pressure, and turbulent quantities through additional drift terms in the corresponding equations. Opposed to previous LES/RANS methods, fluctuations in the LES are not suppressed, thus this approach leads to clean conditions at the LES/RANS interfaces. The results demonstrate that our hybrid solver leads to significantly improved results with moderate computational overhead compared to traditional LES, making it a promising candidate for industrial flow simulations.
Data-Driven Turbulence Modeling
An efficient data assimilation (DA) approach based on the discrete adjoint method for RANS simulations was devised. It allows to tune turbulence modeling parameter fields such that available (typically sparse) measurement data is matched by the simulation results, while specified regularization constraints remain fulfilled. The proposed approach allows to combine the good sides of both experiments and simulations. Stand-alone, the former provide accurate data, which often are sparse, however, and the latter leads to spatially coherent fields, but in general with significant uncertainties. It has been demonstrated that combined within our DA approach, accurate field solutions can be achieved, which is very promising.
Development of PDF Solution Algorithms
Compared with RANS models, PDF methods are computationally more expensive and challenging. Due to its high dimensionality, the PDF transport equation is solved by a particle method [external page Jenny and Meyer 2011]. We have been working on the development of computationally efficient and robust solution algorithms for joint velocity-frequency-composition PDF methods [external page Jenny et al. 2001a and external page 2001b].