Bio Fluid Dynamics, Biomedical Optics
Fluid dynamics in biological systems is a research area, which is mainly driven by questions related to life sciences. In many cases, the background of biologists and medical doctors is not suited to investigate complex transport processes of various fluid compositions, which often are crucial for a deep understanding of the problems at hand. Therefore, bio-fluid dynamics is a very interdisciplinary field, which necessarily involves tight collaborations between life scientists, physicists and engineers.
Modeling the Flow in the Cerebral Microcirculation
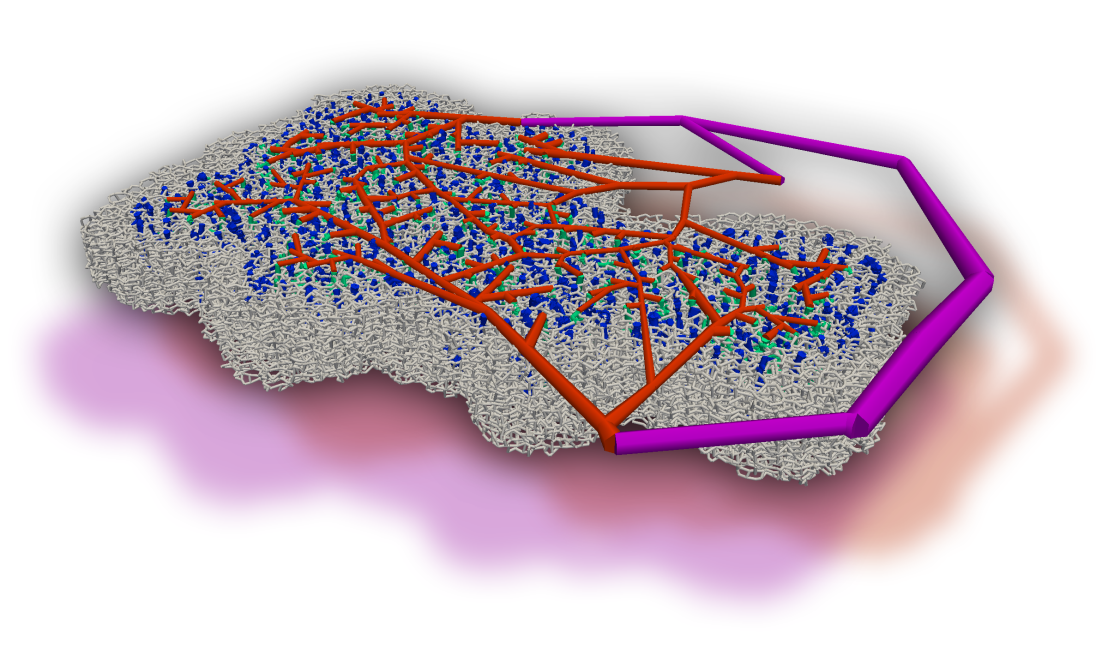
Neurovascular coupling describes the response of the vasculature to neuronal activation. It is well known that, in order to increase the flow rate, the corresponding penetrating arterioles are dilated, which changes the supply of oxygen and metabolites locally. Recently, evidence was found which supports the hypothesis that further regulation mechanisms play a role on a smaller scale. In our research, we focus on the effect of the vasculature in regulating blood flow during health and disease, and on deriving mechanisms that explain phenomena observed in vivo, such as the homogenization of flow in the activated region, which could be caused by a convenient re-distribution of red blood cells [external page Schmid et al. 2015].
We developed a model which tracks red blood cells (RBCs) individually and is capable of capturing transient effects. Our model accounts for the Fahraeus, the Fahraeus-Lindqvist and the phase-separation effect. The definition of appropriate boundary conditions constitutes one of the major challenges for modeling the flow in realistic microvascular networks. Therefore, Schmid and coworkers [external page 2017] introduced a hierarchical approach, which embeds a realistic implant into a large artificial network.
In collaboration with Prof. Bruno Weber (external page University of Zurich), we used simulations to improve our understanding of the most relevant anatomical and functional features of the cortical vasculature [external page Schmid et al. 2019a]. We could show that small-scale regulations, caused by the impact of individual RBCs on the flow field, contribute to the overall robustness of the perfusion [external page Schmid et al. 2019b]. Furthermore, we observed that the severity of micro-strokes is strongly affected by the local vascular topology and baseline flow field [external page Schmid et al. 2021].
To further improve our understanding of the relevant blood regulation mechanisms, we developed an inverse modeling approach which predicts how blood vessels optimally should adapt to achieve desired flow distributions. Our results suggest that diameter changes of capillaries and larger blood vessels are necessary to obtain a localized increase in blood flow [external page Epp et al. 2020].
Oxygen Transport in the Microcirculation
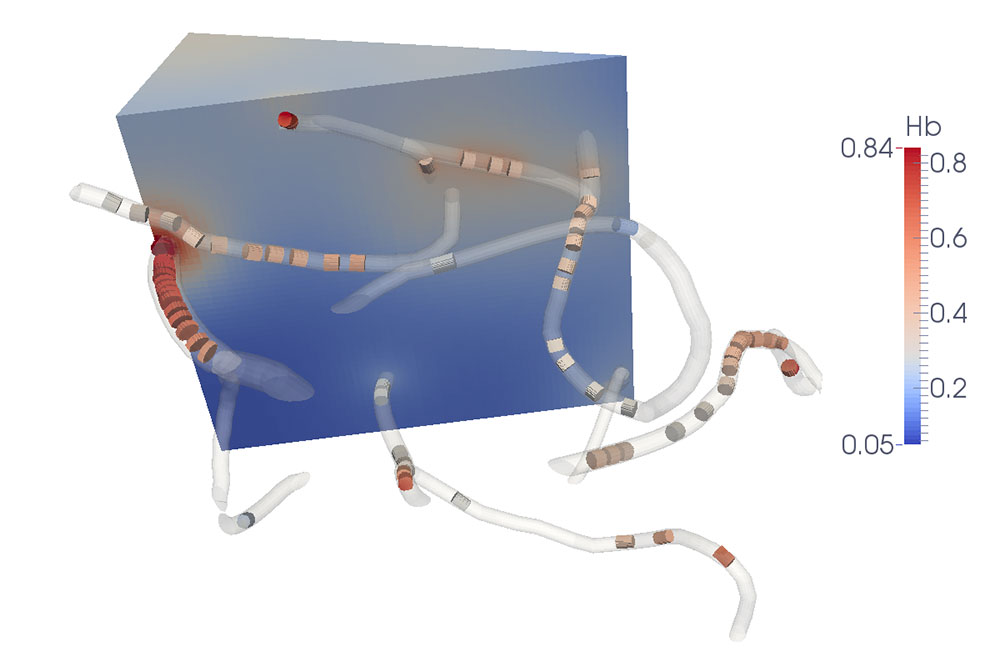
The most fundamental function of the blood circulation is oxygen transport to the tissue. Gas and nutrient exchange mostly occurs at the capillary level due to their large total surface area. In organs such as the brain or in muscles, oxygen supply is a dynamic process that is strongly linked to the metabolism. The mechanisms involved in this coupling are only partly understood, especially at capillary level, but are of fundamental importance in medical imaging and disorders such as Alzheimer’s disease.
In collaboration with Prof. Bruno Weber (external page University of Zürich), a new model for oxygen transport from capillaries to the tissue has been developed [external page Lücker at al. 2014]. The presence of individual moving red blood cells in this model allows us to capture the spatial and temporal heterogeneity of blood flow and its influence on tissue oxygenation [external page Lücker et al. 2017]. Subsequent model refinements and applications in realistic capillary networks [external page Lücker et al. 2018a and external page 2018b] have documented a strong reduction of the heterogeneity in tissue oxygen supply due to diffusive transfer among red blood cells within single capillaries as well as across capillaries. These results provide new insights into the effects of cerebral small vessel disease on tissue oxygenation and brain function. Read more
Modeling Cardiac Mechanics
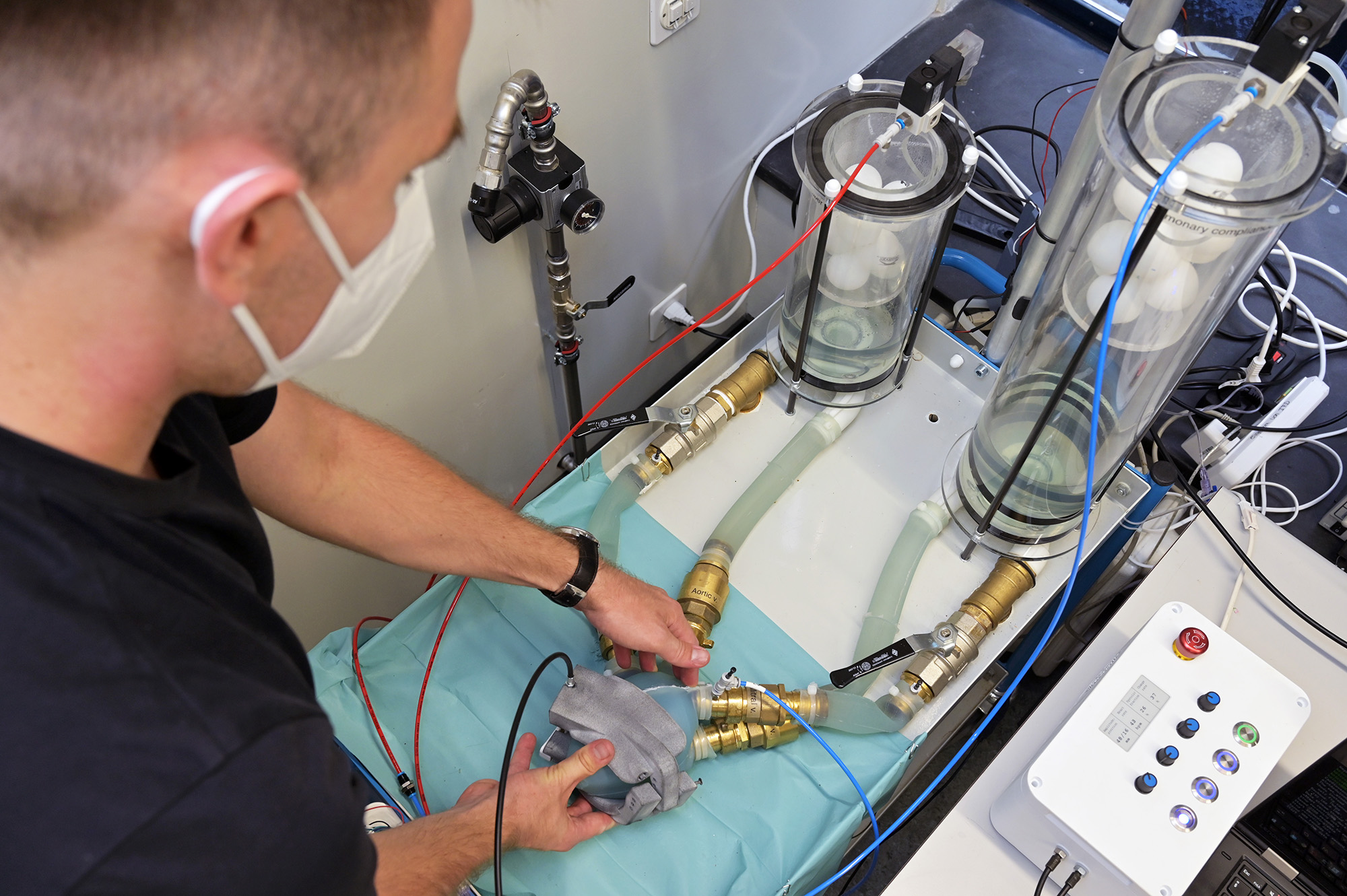
The heart is a sophisticated system: only little damage to its structure, excitation system or other parts can affect the pumping effectiveness severely and cause serious health risks. In fact, the leading cause of death in Switzerland has been heart failure for several years. Medical devices can assist insufficiently working hearts to pump blood. However, the design and optimization of such devices is complicated by a lack of parameter data and limited experimental capabilities. Here, computational models can step in to gain a better understanding of the fluid mechanics of the heart.
We develop a fluid-structure-interaction model which allows for testing and optimization of medical devices. The structure of the heart is represented by a finite element model whereas the blood is modeled by a zero dimensional hydraulic circuit. Both models are coupled implicitly. By imposing boundary conditions on the pericardium we can investigate how assistive devices influence the contraction and cardiac output.
The model is mainly thought to be used for the development of assistive devices which act directly on the heart's surface. However, other applications are conceivable as well. In addition to our numerical simulation efforts, we have recently started complementing these with experimental studies (see figure).
Biomedical Optics and Salmonella Dynamics
Sormaz, Jenny, and coworkers [external page 2009, external page 2010a, external page 2010b, external page 2012, and external page 2015] developed an efficient Monte Carlo algorithm and simulator for scattering of polarized light, which has been employed to investigate improved methods for biomedical optics. The same simulator was also applied to study Salmonella dynamics in collaboration with Prof. Wolf-Dietrich Hardt’s group from the Institute of Microbiology, ETH [external page Winnen et al. 2008] and [external page Misselwitz et al. 2012].